LipidBrick® is a novel range of proprietary cationic lipids dedicated to the formulation of LNPs for the development of mRNA-based vaccines and thera...
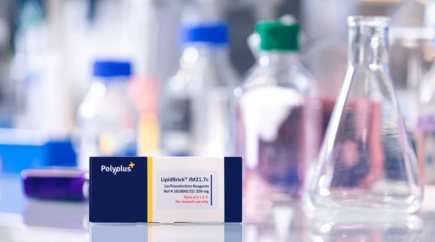
Historically, RNA has been used to uncover the genetic basis and details of specific biological mechanisms. During the last three decades, the potential for RNA to serve as a therapeutic modality has been recognized and early successes achieved. There are challenges to the delivery of RNA, however. Various approaches have been developed for both in vitro and in vivo applications. Lipid-based solutions have been used in approved products, but issues with specificity and stability remain that continue to be the focus on innovative research.
![]() |
Claire Gueguen Ph.D., R&D Life Science Manager at Polyplus®. |
![]() |
Malik Hellal Ph.D., Senior Scientist Chemistry at Polyplus®. |
|
Maria Nicla Loviglio Ph.D., Scientific Support Specialist at Polyplus®. |
![]() |
Patrick Erbacher Ph.D., Chief Scientific officer at Polyplus®.
|
![]() |
Alengo Nyamay’antu, Ph.D, Scientific Marketing & Communication leader at Polyplus®.
|
Ribonucleic acid (RNA) in its various forms helps translate information encoded in deoxyribonucleic acid (DNA) into cellular functions via the production of various proteins and enzymes. Messenger RNA (mRNA), transfer RNA (tRNA) and ribosomal RNA (rRNA) comprise the three major classes.1
Unlike DNA, RNA is relatively short-lived due to degradation by RNase enzymes. On the positive side, RNA does not need to enter the nucleus to initiate protein production. For this reason, RNA – both mRNA and anti-sense RNA (RNAi), which binds to specific RNA molecules to inhibit their activity – has been extensively used by researchers to uncover the genetic basis and details of specific biological mechanisms, information that has increased understanding of many diseases and their causes.
RNA interference was first discovered in 1990 when scientists observed that particular RNA strands had the ability to suppress gene expression in plants.2 This discovery catalyzed extensive research focused on understanding RNAi mechanisms of action, leading to the identification of different types of RNA, including small interfering RNA (siRNA), a small non-coding, double-stranded RNA that is generally complementary to the coding region of a target mRNA; microRNA (miRNA), small non-coding RNA molecules that regulate the expression of multiple mRNAs by blocking translation or promoting degradation; and short/small hairpin RNA (shRNA), synthetic RNA strands with tight hairpin turns that can be used for gene silencing.
The blockage of post-transcriptional translation by small pieces of RNA to silence particular genes or sets of genes is a natural process in normal cell regulation. By targeting specific RNA sequences, researchers can determine which genes or sets of genes control the expression of specific proteins. This knowledge has been applied to the development of RNA-based therapeutics.1,3
RNAi therapies, several of which have been approved by the US Food and Drug Administration (FDA), are based on short single-stranded oligonucleotides (antisense oligonucleotides, or ASOs) that are complementary to a certain region of a target RNA (such as an mRNA).4 A couple of FDA-approved RNA treatments are based on siRNA, such as Patisiran (marketed as Onpattro) and Givosiran (marketed as Givlaari)5. One RNA aptamer drug – a short single-stranded nucleic acid that can bind a specific protein, peptide, carbohydrate, or other types of molecules depending on its tertiary structure – has also been approved by FDA, under the name of Macugen (pegaptanib).
Increasing numbers of RNA interference therapies and vaccines are also progressing rapidly through clinical development, including those based on ASOs, siRNAs, and miRNAs, among others.5,6
In addition to RNA technologies that directly interfere with expressed mRNA or mRNA expression, blocking protein production, there are those that cause the expression of target proteins whose deficiencies can lead to disease.
Exogenous delivery of coding synthetic mRNA has of course been leveraged for COVID-19 vaccines and is being developed for many other antiviral and anticancer vaccines and cancer immunotherapies progressing through clinical studies.7-14 Newer candidates leverage self-amplifying mRNA to enable reduced doses and dosing frequencies.15 RNA is also being explored in the fields of regenerative medicine, in vivo delivery of gene-editing agents, gene therapy, and for the reprogramming of cells for research and therapeutic applications.4,16-18 Compared to plasmid DNA, RNA therapeutics and vaccines provide transient expression in the cytoplasm. There is no requirement for delivery into the nucleus and no risk of insertional mutagenesis.4,8 mRNA vaccines, meanwhile, can be rapidly designed and manufactured using platform processes.20
In addition, unlike small-molecules drugs, which are limited to interacting with the small percentage of proteins that have active binding sites (known as “druggable targets”)2, RNA as an active therapeutic or immunological agent modulate protein production by leveraging existing cellular biochemistry3. Similarly, biologic drugs such as recombinant proteins and antibodies need to interact with proteins that have already been expressed, and also have the correct structure, folding properties, and post-translational modifications to do so.
Furthermore, to produce RNA therapeutics and vaccines only requires the target nucleotide sequence, whether that is a malfunctioning mRNA or a viral protein.3 The active drug substance or vaccine active can then be quickly synthesized. There is no need for the development of the specialized cell lines and cell culture/fermentation processes required to produce recombinant proteins or establishment of the complicated synthetic routes necessary to manufacture structurally complex chemical APIs, both of which are time- and cost-intensive.
Market estimates for RNA therapies and vaccines are generally presented separately for RNA antisense/interference and mRNA products. Global revenue for the former in 2021 is reported to be nearly $5.88 billion and expanding at a compound annually growth rate (CAGR) of 18.68% through 2032.21 The value of the market is projected to surpass $6.99 billion by the end of that year.22 Drivers include the applicability to treat both common and rare diseases, the specificity and safety of RNA-based medicines, growing use of RNA molecules for gene-editing applications and the expansion of many DNA-focused companies into the RNA sector.
The value of the global market for mRNA therapeutics and vaccines is estimated to reach $128.14 billion by 2030.23 Not surprisingly, by application, the infectious diseases segment accounts for the highest revenue currently, as does the prophylactic vaccines segment by type. In addition to the success of the COVID-19 mRNA vaccines, other growth drivers include the rising prevalence of long-lasting infectious diseases, advances in stabilization and delivery technologies for mRNA, and the ability to use mRNA therapeutics as personalized medicines. According to one market research firm, as of late October 2022, more than 35 companies were evaluating over 195 mRNA therapeutic and vaccine candidates at different stages of development for a myriad of disease indications.24
The delivery of RNA to cells both in vitro and in vivo is…