Available at research and GMP grade to intensify production of recombinant AAV rAAV viral vectors at any scale from benchtop to 2000L scale bioreactor...
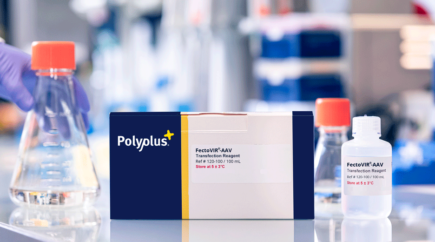
Authors:
1 Process Development, Lonza Houston Inc., 14905 Kirby Dr, Houston, TX, 77047
2 Research & Development, Lonza Houston, Inc. 14905 Kirby Dr, Houston, TX 77047
3 Applied Statistics, Lonza, 101 International Dr., Portsmouth, NH, 03801
With at least two dozen gene therapies having received marketing authorization around the world and up to eight more with the potential to receive regulatory approval in the U.S. in 20231, treatments based on genetic modification have established a strong foothold globally. Hundreds more gene and gene-modified cell therapy candidates are under investigation in over 1000 clinical trials1, creating high expectations for continued growth of the field.
Direct gene therapies account for approximately one-third of ongoing clinical trials1, and three-quarters of the candidates being investigated in those studies rely on delivery of the genetic material using adeno-associated viral (AAV) vectors2. That is because AAV vectors are non-pathogenic, elicit minimal undesired immunogenic reactions and various wild-type and engineered AAV serotypes are available for targeting different tissue and cell types, allowing their use for the treatment of a multitude of indications.
A key challenge for direct gene therapy developers today is the efficient and cost-effective large-scale production of AAV vectors. Traditional lab-scale adherent cell-culture processes are not readily scalable nor practical to implement in a GMP environment. Typical processes for AAV production also generally suffer from low yields and require the use of costly raw materials. As a result, the cost of goods for gene therapy manufacturing ranges between $500,000 and $1 million3, which if not addressed will prohibit commercialization of gene therapies for the treatment of more prevalent diseases and larger patient populations.
Physical scaling of manufacturing processes is only one part of the needed solution. Improving processes efficiency and productivity to lower the cost-per-dose is essential. Process optimization and intensification must be realized in combination with the development of more readily scalable processes. Process intensification can be achieved using fit-for-purpose plasmids and transfection reagents. A design-of-experiment (DoE) approach, meanwhile, enables rapid identification of optimum process parameters.
Transient transfection is a complex, multi-step process that involves incorporation of multiple DNA plasmids into HEK293 cells. Careful design of key reagents can have a direct impact on process performance. Plasmid engineering, for instance, can ensure that DNA used in transfection processes contains only the necessary genetic material and is of high purity, eliminating potential hindrances to effective transection and capsid formation. Similarly, transfection reagents designed for AAV production, and even more specifically for suspension-based AAV manufacturing, provide more stable plasmid-reagent complexes, reduced complexation volumes and higher transfection efficiencies.
One example of the latter is FectoVIR®-AAV transfection reagent from Polyplus-Part of Sartorius, which was specifically designed to enable scalable, suspension-based AAV vector production via transient transfection. In addition to providing a two-fold increase in AAV titers compared to Polyplus standard, polyethylene imine (PEI) reagent PEIpro® and a 10-fold increase in titer compared to other PEI-based reagents on the market, FectoVIR-AAV transfection reagent exhibits this improved performance at complexation volumes of 5% (and in some cases as little as 1%) of the culture volume, compared to the typical 10%. Complexes of the reagent and plasmid DNA are also much more stable, allowing longer transfer times if necessary for more fragile complexes. Consistent performance is observed at small to large scale, with availability from research to GMP grade.
The productivity and efficiency of AAV capsid formation are influenced by many different process parameters, which often vary depending on the specific serotype and nature of the GOI. A deep understanding of the process is necessary to optimize the numerous factors that can influence yield, quality, and other performance attributes. That level of understanding can be gained more rapidly using a DoE approach, which serves as a systematic and efficient mechanism for gaining insight into the critical process parameters (CPPs) that directly influence critical quality attributes (CQAs) while also revealing important interactions between those CPPs and potential non-linear effects.
Using a DoE approach during process development of AAV manufacturing processes leveraging triple transient transfection therefore results in scalable processes with well-defined operating ranges and optimized performance with respect to cell growth and viability, productivity (titer), packaging efficiency (percentage full capsids) and functionality. In addition to plasmid design and choice off transfection reagent, CPPs may include the total plasmid quantity, plasmid ratio, plasmid/transfection reagent ratio, DNA/transfection reagent complexation time and volume, medium, cell line and cell density, temperature, pH and dissolved oxygen.
Relevant outcomes for AAV manufacturing processes evaluated during DoE studies include functional and viral genome titers, percentage full capsids, the presence of encapsulated DNA and host-cell DNA impurities, other product-specific CQAs, and cost-per-dose. The goal is to identify the process conditions that afford optimized performance with respect to titer, quality, and cost.
Successful DoE studies begin with a clear definition of the study goals and selection of the appropriate factors for evaluation based on previous experience with transient transfection processes, considering resources, capabilities, and timelines. The best experimental plans minimize variability and leverage analytical methods that enable consistent and reliable evaluation of the target outputs. Confirmation through iterative studies with expanded operating ranges helps ensure full coverage of the design space. With this approach, AAV vector yields can often be increased drastically in just a few months, providing purer products that require simpler downstream processing, leading to dramatically reduced costs and often shorter run times.
Packaging efficiency (percent full capsids) is an important CQA for AAV- manufacturing processes. Analysis of the literature and internal Lonza data indicate that upstream crude harvests following triple transfection process to produce AAV vectors contain approximately 5 to 50% full AAV capsids depending on the serotype and GOI. This low percentage full creates real challenges for downstream purification of AAV vectors and presents an opportunity for improvement that could have significant impacts on the quality and cost of AAV-based gene therapies.
To identify CPPs that influence this crucial CQA, a DoE approach was used to evaluate the impact of several process parameters on both AAV titer and packaging efficiency using the Ambr 250® High-Throughput Platform (Sartorius). Proprietary Lonza packaging plasmids (RepCap and helper) and AAV8-GFP (green fluorescent protein) as the GOI were employed for the study model. FectoVIR-AAV was employed as the transfection reagent. AAV titer (Vg/mL) and percentage full capsids were measured for upstream crude harvests using droplet digital polymerase chain reaction (ddPCR) and size-exclusion chromatography (SEC) coupled with ultraviolet (UV) and multi-angle light scattering (MALS) detectors, respectively.
The DoE study was implemented in two steps. In the first set of experiments, the effect of varying the ratio of the RepCap and helper plasmids and GOI was explored. Twelve different plasmid ratios were investigated, with transient transfection performed in both shake flasks and Ambr 250 mini-bioreactors. Notably, for all 12 plasmid ratios, higher titers were observed for productions performed in the mini bioreactors, while the percentage of full capsids was similar or higher in the bioreactors vs. the shake flasks (Fig1).
More specifically, three different plasmid ratios clearly provided higher titers, while two different plasmid ratios afforded slightly more full capsids. The results indicated that the plasmid ratio plays an important role in the productivity and packaging efficiency of AAV transient transfection reactions.
In addition, the DoE analysis led to the establishment of strong models for the titer and percentage full capsids expected as a function of the plasmid ratios. A Prediction Profiler was used to graphically represent both models and predict the optimal conditions for maximizing both titer and percentage full capsids. Actual and predicted log titer (vg/mL) values when plotted against one another provided a linear graph with a root mean square error value of 0.1984 and a P value < 0.0001. Similarly, actual vs. predicted percentage full values yielded a linear graph with an RMSE of 0.046 and a P value also < 0.0001. This data was used to identify an optimal plasmid ratio for AAV8-GFP that maximized both productivity and packaging efficiency (Fig 2).
This plasmid ratio was then used in the second step of the DoE study, which involved evaluation of several process parameters [temperature, pH, elapsed time to shift the setpoint for pH and Temperature after transfection (0, 6 and 24 hours) and quantities of glucose and Glutemax supplements]. All runs were performed in Ambr 250 mini-bioreactors, with viral titer and percentage full values determined using ddPCR and SEC-MALS, respectively. The DoE analysis provided strong models for both productivity and packaging efficiency, with respective RMSE values of 0.121657 and 0.023604. Temperature, elapsed time after transfection and pH were found to have the most significant impact on the performance of the AAV transient transfection process.
Prediction Profiler was used to graphically represent both models and predict the optimal conditions for maximizing tier and the percentage of full capsids. In this case, an inverse correlation between productivity and packaging efficiency was observed. It was therefore necessary to find a balance between these two outputs. Based on the model, optimal settings were identified that would provide a packaging efficiency of 69.9%.
Using a DoE approach involving two rounds of experiments, the percentage of full AAV capsids for AAV8-GFP in the crude harvest was increased significantly—by approximately three-fold from the starting baseline (~20% to ~70%). The plasmid ratio, along with temperature, elapsed time after transfection and pH were found to play important roles in determining both productivity and packaging efficiency for the manufacture of this AAV serotype/GOI combination (Fig 3). The DoE data was used to construct a strong model for prediction of optimal conditions that enable maximum AAV packaging efficiency for AAV8-GFP. This approach can readily be implemented into upstream process development activities at Lonza for different AAV serotypes and client specific GOIs.
Gene therapy developers and CDMOs involved in AAV manufacturing are faced with the challenge of developing more efficient and productive processes that provide consistent, high performance from the lab to commercial scale. By working together using a thoughtful DoE approach and purpose-built resources, CDMOs, gene therapy developers and suppliers can all ensure that the industry is able to upscale AAV transient transfection processes more effectively from the start of each process.
___________________________________________________________________________________________________________________________________________________________________________
References