jetOPTIMUS® is an innovative cationic nanotechnology developed to improve DNA transfection efficiency in hard-to-transfect cells.
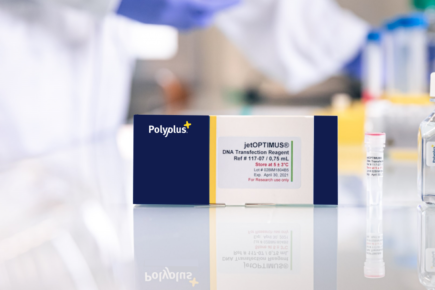
Stem cells are regarded as the most powerful and flexible cell type available to model and repair cellular function. Indeed, stem cells are currently employed in drug-screenings, cell therapies, disease modelling, toxicology and genome editing studies in almost any field of basic and translational research1. Importantly, many of those technologies rely on the successful introduction of genetic and/or protein-based material into stem cells, such as in CRISPR/Cas9 gene editing. However, it is well known that stem cells, particularly pluripotent (i.e. cells able to generate any somatic cell type) are exceptionally difficult to transfect2. The biggest challenge when transfecting stem cells is cell death which has been reported after both non-viral and viral transfection delivery methods(2). Furthermore, as a result of both susceptibility to cell death and fast self-renewal, transfection efficiency can be lower with stem cells than with other cell types.
Researchers manage these challenges by evaluating different delivery methods based on the final requirements of their application, being that a permanent or transient transfection, as well as on a balance of cost/time benefits. Clearly, one size does not fit all when it comes to hard-to-transfect stem cells. For example, it is established that for permanent expression of large genetic material the AAV vector system is optimal. Equally, when cell suspension and longer post-recovery time is possible, nucleofection is preferred. However, when fast, transient, economical transfection of adherent cells is required, especially in high-throughput and proof-of-concepts screenings, a chemical-based delivery would be ideal. Notably, a transient, economical and non-toxic transfection reagent could also be used in diagnostics, especially with CRISPR-cas9 being proposed as a precise and alternative diagnostic tool of infection diseases, such as SARS-CoV-23.
In order to unleash the benefits of genetic manipulation with a chemical-based transfection, the ideal reagent would need to achieve high transfection efficiency (in the case of stem cells above 50%) while minimising any cell viability issues common with stem cells. Here we report the transfection efficiency performance of jetOPTIMUS®, a chemical-based transfection reagent, in three hard-to-transfect stem cells: mesenchymal stem cells (hMSCs), mouse embryonic stem cells (mESCs) and human induced pluripotent stem cells (hiPSCs). Furthermore, we assess the transfection efficiency of jetOPTIMUS® on hPSCs cultured on several commercially available and commonly used culture and substrate systems.
Here we demonstrate that jetOPTIMUS® achieves stable transfection efficiency in primary hMSCs and ever higher efficiency in delicate mouse and human pluripotent stem cells, without inducing cell death when tested. Moreover, we report a list of commonly used hiPSCs culture media and substrates that are best suited for transfection in combination with jetOPTIMUS®. In conclusion, jetOPTIMUS® transfection reagent demonstrates robust, non-toxic and efficient gene expression delivery in hard-to-transfect primary hMSCs, mESCs and induced pluripotent stem cells.
Human Mesenchymal Stem Cells (hMSCs) (#PT-2501, Lonza Bioscience) were cultivated in MSCGM™ BulletKit™ (#PT-3001, Lonza Bioscience) containing MSCBM™ Basal Media (#PT-3238, Lonza Bioscience) supplemented with MSCGM™ SingleQuots Supplement Kit (#PT-4105, Lonza Bioscience). HMSC were seeded at 6 000 cells per cm² in a T-75 cm² flask, cultivated in a static incubator at 37°C with 5% CO2 and split twice a week with Trypsin/EDTA solution (#CC-5012, Lonza Bioscience).
Murine Embryonic Stem cells (mESs) (#NBP1-41162, Novus Biological) were cultivated in DMEM 4.5 g/L glucose (#D5671, Sigma-Aldrich) supplemented with 15% FBS (#S1810-500, Biowest), 1% Penicillin/Streptomycin 10K (#P4333, Sigma-Aldrich), 1% Non-Essential Amino Acids (#BE13-114E, Lonza Bioscience), 1% L-Glutamin 200 mM (#G7513, Sigma-Aldrich), 0.2% mLIF (#8878-LF-025, Novus Biologicals) at 5 mg/mL and 1% EmbryoMax™ 2-Mercaptoethanol 100X (#ES-007, Sigma-Aldrich) in a T-75 cm² flask coated with 10 mL of sterile 0.1% Gelatin solution (#G1890, Sigma-Aldrich) prepared at room temperature for 1 hour. Cells were seeded at 4 000 cells per cm², cultivated in a static incubator at 37°C with 5% CO2 and split twice a week with CTS™ TrypLE™ Select Enzyme (#A1285901, Gibco) at 37°C with 5% CO2 for 3 to 5 minutes.
Human Induced Pluripotent Stem Cells (hiPSCs) from Fibroblast (#ASE-9101, Applied StemCell) were cultivated in mTeSR™ 1 medium (#85850, STEMCELL™ Technologies) on a 6-well plate coated with Corning® Matrigel® Matrix. Colonies were passaged by gentle manual scraping once a week in hiPSCs media in the presence of 10 µM of ROCK inhibitor (#ACS-3030, ATCC) for 24 hours. Medium was refreshed every two days. In order to plate hiPSCs for transfection, colonies were dissociated as single cells as follows. Colonies were washed with 2 mL of pre-warmed medium with 10 µM of ROCK inhibitor. The medium was removed and 1 mL of Accutase™ (#07920, STEM CELL™ Technologies) was added per well. Cells were incubated 3 to 10 minutes at 37°C with 5% CO2 and removed from plate with 1 mL of pre-warmed medium. Cells were centrifuged at 200 g during 5 minutes and resuspended in 0.2 mL of complete medium per well collected ready for cell count.
Alternative stem cell maintenance media were used for selective efficiency experiments as follows: TeSR™-E8™ (Stemcell™ Technologies; E8™ medium (Thermo Fisher Scientific); StemFlex™ (Thermo Fisher Scientific); PluriSTEM™ Human ES/iPS cell (EMD Millipore™) and StemMACS™ iPS Brew (Miltenyi Biotec). Alternative stem cell matric coating were used for selective efficiency experiments as follows: Matrigel®-hSC qualified (Corning®); Vitronectin (Thermo Fisher Scientific) and Geltrex™ (Thermo Fisher Scientific).
The plasmid pCMV_eGFP_Luc (6.4kb) coding for a protein fusion enhanced Green Fluorescent Protein (eGFP)/luciferase with a cytomegalovirus (CMV) promoter was purchased from Clontech (#6169-1). The plasmid pEF1a_eGFP (4.9 kb) coding for eGFP with a Elongation Factor-1 alpha (EF1a) promoter was purchased from Ezyvec (#A626.1). pCMV_eGFP_Luc was used to transfect hMSC and mES and pEF1a_eGFP was used to transfect hiPSC.
At 72 hours prior to transfection, hMSCs were seeded in a 24-well plate at 12 000 cells per well in 500 µL of corresponding cell maintenance media and incubated at 37°C with 5% CO2. For mESCs, 24 hours prior to transfection, cells were seeded in a 24-well plate at 20 000 cells per well in 500 µL of mES media and incubated at 37°C with 5% CO2. For hiPSCs, 24 hours prior to transfection, cells were seeded in a 24-well plate coated with Corning® Matrigel® Matrix containing 2 mL of mTeSR medium with 10 µM of ROCK inhibitor at 100 000 cells per well and incubated at 37°C with 5% CO2.
For hMSCs and hiPCSs, 30 minutes before transfection, the culture medium was removed and replaced by 500 µL of MSCBM™ without supplement or 500 µL of hiPSCs media with 10 µM of ROCK inhibitor, respectively, and the plate was returned to incubator.
DNA transfection was carried out with jetOPTIMUS® (#117-01, Polyplus Transfection). Transfection reactions were prepared as follows: 500 ng of plasmid was diluted in 50 µL of jetOPTIMUS® Buffer (Polyplus-Transfection) and vortexed briefly. Next, 0.5 to 0.75 µL of jetOPTIMUS® for hMSCs and mES cell lines or 0.75 µL of jetOPTIMUS® for hiPSCs was added on the diluted plasmid. After 1 second of vortexing, the solution was incubated at room temperature during 10 minutes. Next, 50 µL of jetOPTIMUS®/plasmid complexes solution were added onto the cells. Plates were returned to the 37°C and 5% CO2 incubator. After 4 hours, the medium was removed and replaced by 500 µL of culture medium for hMSCs and mESs or the medium was diluted with 500 µL of culture medium for hiPSCs. Plates were returned to the 37°C and 5% CO2 incubator. Cells were incubated for 24 h prior to flow cytometry.
Cellular density and viability were assessed by Trypan Blue staining (#T8154, Sigma-Aldrich) and counted with a TC-20 Automated Cells Counter (#1450102, Bio-Rad).
Cells were washed with 0.5 mL of sterile PBS 1X. After removing the PBS, cells were detached with 100 µL of Trypsin/EDTA solution for hMSC, 100 µL of CTS™ TrypLE™ Select Enzyme solution for mES or 100 µL of Accutase™ for hiPSC during 3 to 10 minutes at 37°C with 5% CO2. 400 µL of TNS (hMSC), culture medium (mES) or PBS 1X with 2% FBS and 2 mM EDTA (hiPCS) with propidium iodide at 1 µg/mL were added into the well. A sum total of 200 µL were transferred in a 96-well U-bottom plate and read on flow cytometer Guava EasyCyte 5HT (Luminex) and analysed with GuavaSoft and InCyte Software.
1. Liu G, David BT, Trawczynski M, Fessler RG. Advances in Pluripotent Stem Cells: History, Mechanisms, Technologies, and Applications. Stem Cell Rev Rep. 2020;16(1):3-32. doi:10.1007/s12015-019-09935-x
2. Yamoah MA, Thai PN, Zhang XD. Transgene Delivery to Human Induced Pluripotent Stem Cells Using Nanoparticles. Pharmaceuticals (Basel). 2021;14(4):334. Published 2021 Apr 6. doi:10.3390/ph14040334
3. Kaminski MM, Abudayyeh OO, Gootenberg JS, Zhang F, Collins JJ. CRISPR-based diagnostics. Nat Biomed Eng. 2021;5(7):643-656. doi:10.1038/s41551-021-00760-7
Thibaut Benchimol, Alengo Nyamay’antu, Claire Gueguen